Are Engineered Small Proteins the Next-generation Immune Checkpoint Inhibitors?
The inaugural International Cancer Immunotherapy Conference, hosted jointly by the Cancer Research Institute (CRI), the Association for Cancer Immunotherapy (CIMT), the European Academy of Tumor Immunology (EATI), and the American Association for Cancer Research (AACR), began Wednesday, September 16, at the Sheraton New York Times Square Hotel. This year’s conference theme is “Translating Science Into Survival.”
A study presented by Stanford researchers at the conference may take immunotherapy to the next level.
Anyone who follows the progress made with cancer immunotherapy will have gleaned the success the cancer research community has had in developing, testing, and getting FDA-approved immune checkpoint inhibitors to the clinic.
Cancer cells often evade immune recognition by engaging the PD-1/PD-L1 pathway, a mechanism by which they apply “brakes” on immune cells called T cells, preventing the T cells from attacking cancer cells. Immune checkpoint inhibitors, such as pembrolizumab (Keytruda), the immunotherapy former U.S. President Jimmy Carter is being treated with, block the PD1/PD-L1 pathway and release the brakes on T cells so that they can recognize and attack cancer cells. Owing to successful outcomes from clinical trials, the FDA has approved three immune checkpoint inhibitors so far—pembrolizumab and nivolumab (which block PD-1), and ipilimumab (which blocks another checkpoint, CTLA4)—to treat certain metastatic melanomas and advanced lung cancers. All current FDA-approved immune checkpoint inhibitors are antibodies.
But are antibodies truly the best immune checkpoint inhibitors?
That’s a question raised by scientists in the laboratory of Irving Weissman, MD, at the Ludwig Center at Stanford University School of Medicine in California. “This question, at this point, remains unanswered and even unaddressed,” says Aaron Ring, an MD/PhD student from Weissman’s lab. In addition to being scientifically intriguing, this question holds the very exciting potential to create even better therapeutics for patients, adds Ring, who will shortly be taking up a position as assistant professor in the Department of Immunobiology at Yale University School of Medicine.
Sydney Gordon, a graduate student in Weissman’s lab, explained that their team is now addressing this question because they recognize that the immune checkpoint-inhibiting antibodies have some overlooked limitations. “First, it would be ideal if drugs that target PD-L1 could get inside the tumors. However, antibodies, because of their large size, cannot get there effectively, which prevents them from working to their full potential. Second, antibodies against PD-1 and PD-L1 can result in counterproductive collateral damage, depleting some of the very antitumor immune cells they are supposed to activate,” she said.
The hypothesis
Gordon, Ring, and colleagues hypothesized that an engineered protein that is much smaller in size than a conventional antibody and targets PD-L1 to block the PD-1/PD-L1 signaling pathway could potentially overcome these limitations and function as a superior immune checkpoint inhibitor. The team indeed developed such a small protein and presented their findings at the CRI-CIMT-EATI-AACR International Cancer Immunotherapy Conference.
Engineering the high-affinity PD-1 protein
The Stanford researchers engineered the high-affinity PD-1 protein by a process called directed evolution, Ring explained. “Our goal was to enhance the ability of the natural PD-1 receptor to bind extremely tightly to PD-L1 as a small competitive “decoy” that is about one tenth the size of an antibody,” he said. The researchers generated more than 100 million variants of PD-1 that contained mutations at the PD-L1 binding interface and within the core of the protein. “By selecting for tight binders through increasingly more difficult conditions, we let the principles of natural selection do the hard work and guide us to the strongest binders. In the end, our best variants bound to PD-L1 about 50,000-times stronger than the natural PD-1 protein,” said Ring.
Testing the small protein’s efficacy
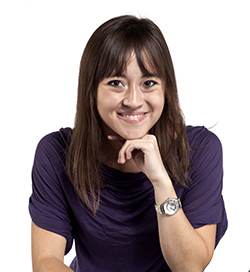
Sydney Gordon, a PhD student at Stanford University, presented the team’s findings at the immunotherapy conference this week.
The next step in their research was to test if the high-affinity PD-1 protein they engineered lived up to expectations.
Through a series of experiments in mice, the researchers first demonstrated that the small protein was more efficient in penetrating the tumors than were anti-PD-L1 antibodies, which went as far as gathering around the blood vessels outside the tumors. Next, they showed that the high-affinity PD-1 protein did not impact T-cell numbers in the blood and lymph nodes collected from mice whose tumors were treated with the protein, as opposed to anti-PD-L1 antibodies, which caused significant depletion of T cells that worsened when they combined the antibody with other immunotherapies.
“This may have important implications when considering how to optimize immunotherapeutic combinations for patients,” Gordon said.
Further studies by the team showed that while both high-affinity PD-1 protein and anti-PD-L1 antibodies were effective in shrinking small tumors, only the high-affinity PD-1 protein was able to slow the growth of large tumors. It was also more effective at shrinking large tumors when combined with an anti-CTLA4-antibody. The anti-PD-L1 antibodies, on the other hand, were ineffective at eliminating large tumors, either alone or in combination with an anti-CTLA4-antibody.
“This was the key study for us that best highlighted a situation where small protein therapeutics could produce a better outcome than conventional antibodies,” Gordon said.
“Given how well anti-PD-1 and anti-PD-L1 antibodies are working for cancer patients, it may seem like a reasonable assumption that we have adequately targeted the pathway at this point. However, our results show that significantly more antitumor activity is possible using a small-protein therapeutic compared with a conventional antibody,” Ring said.
There are, however, some limitations to using the high-affinity PD-1 proteins in patients in their current form, the researchers note. Because of their small size, the high-affinity PD-1 proteins may get excreted from the body more quickly, which means that they would have to be administered more frequently than antibodies. Further, they may be “immunogenic,” meaning patients could develop an immune response to the drug itself. “Both issues would need to be addressed for high-affinity PD-1 or a similar small-protein therapeutic to be developed for clinical use,” Gordon elaborated.
Beyond therapeutic application
The researchers went a step further and evaluated the utility of the high-affinity PD-1 protein in identifying the right patients for this type of therapy.
PD-L1, the target of the high-affinity PD-1 protein, is considered a potential biomarker, in that its presence in a tumor may tell whether or not the patient’s cancer is suitable for immunotherapy targeted toward the PD-1/PD-L1 pathway. However, currently this can only be determined by performing a biopsy of the tumor and studying the tissue under a microscope. There are a couple of problems with this: First, biopsy is an invasive surgical procedure that comes with some risk and discomfort. Second, PD-L1 is not uniformly distributed in a tumor, so there is a possibility of not finding it in the biopsy sample.
So the researchers wondered whether they could measure PD-L1 levels in a noninvasive manner by radiolabeling the high-affinity PD-1 protein, which when injected in a patient will bind to PD-L1 present in the tumor and in metastatic tissues. This could then be imaged using PET. “Together with our collaborators in the Gambhir laboratory we were able to perform in living mice some proof-of-principle studies and demonstrate that it is possible to locate PD-L1 using radiolabeled high-affinity PD-1 protein,” Ring said.
“I would anticipate that many of the advantages that we saw for our engineered PD-1 proteins would extend to other small proteins being developed by other research groups and biotechnology companies,” he added.