Hypoxia and the Lethal Cancer Phenotype: A Conversation with Nobel Laureate Gregg Semenza
In the early 1990s, Gregg Semenza, MD, PhD, was a postdoctoral researcher studying the cellular response to reduced oxygen levels, a state known as hypoxia. His focus was on the erythropoietin gene, EPO, which controls red blood cell production, and thus oxygen delivery. After identifying a sequence within the EPO gene that was critical for the response to hypoxia, Semenza isolated a mystery protein that was bound to this sequence. This newly discovered protein came to be known as hypoxia-inducible factor 1 (HIF-1), which is now understood to be a master regulator of the cellular response to hypoxia. Since the isolation of HIF-1, additional HIFs have been identified as well.
Semenza, now a professor at the Johns Hopkins University School of Medicine and a Fellow of the AACR Academy, was awarded the 2019 Nobel Prize in Physiology or Medicine for his groundbreaking discovery of HIF-1. Semenza shares the prize with William Kaelin, Jr., MD, and Peter Ratcliffe, MD.
Like many cellular processes, the response to hypoxia can be exploited by cancer cells to promote proliferation, spread, and resistance to therapy. We spoke with Semenza about the interplay between hypoxia and cancer, how hypoxia affects responses to existing cancer therapies, and the therapeutic potential of targeting HIFs.
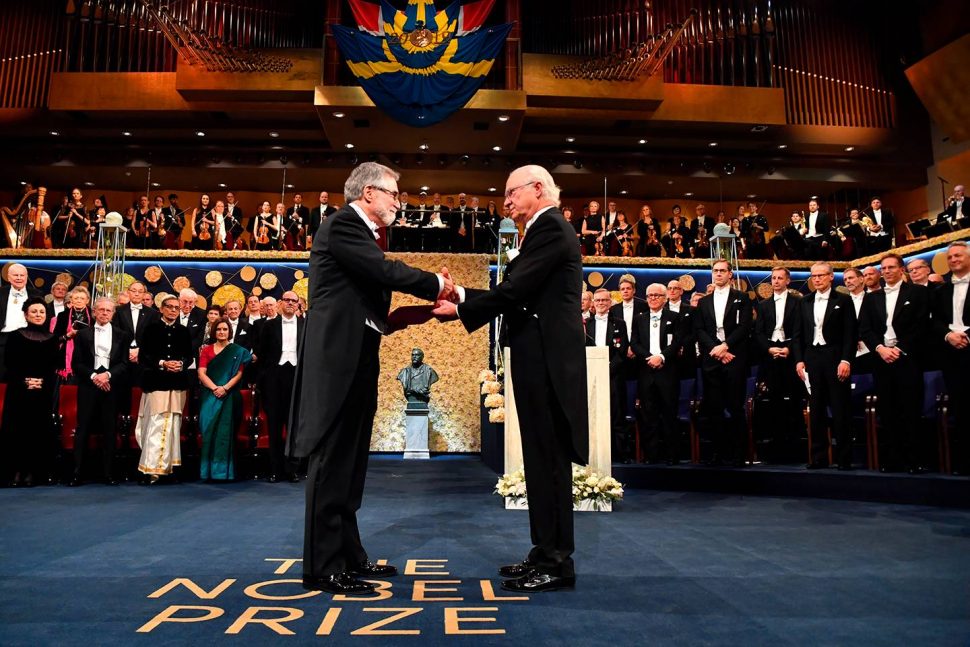
How has the discovery of hypoxia-inducible factors (HIFs) impacted our understanding of the cellular response to hypoxia? What are some of the cellular and physiological processes regulated by hypoxia-inducible factors?
In the years since its discovery, we have learned that HIF-1 is a master regulator that controls the expression of over 4,000 different genes in hypoxic cells. It seems that HIF-1 is critical to just about every cell just about all the time.
One of the processes regulated by HIFs is vascularization. If a cell is not getting enough oxygen, it produces angiogenic factors to stimulate blood vessel growth to bring blood and oxygen. HIFs directly regulate most of the major angiogenic factors, including VEGF, angiopoietins, angiopoietin-like factors, among others.
Another process regulated by HIFs is immune evasion. We and others have identified a number of different genes induced by hypoxia in cancer and immune cells that promote immune evasion. These genes affect both the innate and adaptive immune responses through multiple mechanisms.
How do hypoxia and hypoxia-inducible factors affect cancer progression and prognosis?
Cancers tend to be very hypoxic due to the dramatic surge in oxygen consumption that occurs with the increased proliferation of cells. This turns on HIF activity, which leads to increased vascularization, metabolic adaptation, invasion, metastasis, stem-cell properties, and evasion of the immune system—just about every process contributing to the lethal cancer phenotype that leads to patient mortality.
Of course, the end goal of vascularization in a normal physiologic system is to increase oxygen delivery to the cells. However, in cancers, the blood vessels that form tend to be structurally and functionally abnormal. Thus, even though there is a lot of angiogenic activity within the tumor, the perfusion of the tumor oftentimes remains inadequate to supply sufficient oxygen. In other words, the tumors can be very angiogenic but still remain hypoxic. We know from studies of many different cancers that measurable hypoxia or a very high level of HIF-1 is associated with a much greater incidence of cancer recurrence, metastasis, and cancer-specific mortality.
What are some strategies for measuring tumor hypoxia and using this information to inform treatment or prognosis?
There are a variety of ways that one could measure tumor hypoxia in patient samples. In accessible tumors, it is possible to directly measure O2 levels, for example, by using an Eppendorf microelectrode. Positron emission tomography (PET) scans using 18F-labeled nitroimidazole compounds represent another approach. In addition to detecting hypoxia, immunohistochemistry can be used to detect HIF-1α protein expression in standard tumor biopsy samples. An alternative strategy is to measure the expression levels of HIF target genes using RNA sequencing.
No matter how one measures hypoxia or HIF activity, it’s important to bear in mind that the extent of hypoxia is heterogeneous across regions of the tumor, and HIF activity may also vary from one part of the tumor to another. Because tumor hypoxia is not homogeneous, there is concern that you may get a false idea of tumor hypoxia depending on the part of the tumor that is sampled.
On the other hand, it doesn’t take hypoxia in all parts of the tumor to affect cancer progression; it only takes hypoxia in one part of the tumor to drive invasion and metastasis. This is something that is not well appreciated—the idea that a small subpopulation of cells can have an outsized effect on cancer progression. We need to focus more on some of these subpopulations, as they may be the most important targets for preventing cancer progression.
As far as using measurements of tumor hypoxia to inform treatment, I think we really need a prospective trial in which patients with high HIF levels are treated more aggressively than patients with low HIF levels to understand how this may impact outcomes. It would also be interesting to use a HIF inhibitor under those circumstances, but there aren’t any HIF inhibitors that have been approved for clinical use yet.
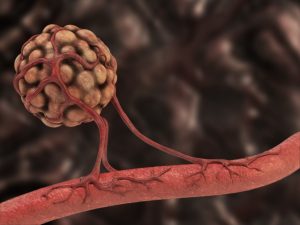
How do hypoxia and HIFs affect the response of tumors to current cancer treatments?
As I’ve mentioned, the tumor’s response to hypoxia typically results in the development of abnormal and poorly functioning blood vessels. Because of this, the tumors remain hypoxic, they make more angiogenic factors, make more blood vessels that are ineffective, and it becomes a vicious cycle that results in very hypoxic cells. So, you might expect that inhibitors of angiogenesis would be a useful therapy; however, the VEGF inhibitors have not been very successful in the clinic.
In animal models, blocking blood vessel growth with VEGF inhibitors leads to more hypoxia in the tumor, which activates the HIF-induced processes that promote metastasis. Of course, the last thing you want from a cancer therapy is to promote metastasis. If this effect also occurs in patients, then it might be useful to administer a HIF inhibitor in concert with a VEGF inhibitor to prevent these countertherapeutic effects. The VEGF inhibitor would make the cancer hypoxic, and the HIF inhibitor would prevent the cancer from adapting to the hypoxia. Experiments in animal models have suggested that this strategy might be useful therapeutically.
Other cancer treatments, such as androgen deprivation therapy for prostate cancer, have also been shown to increase hypoxia within cancer cells. The same dynamic may be occurring, and it may be helpful to administer HIF inhibitors in concert with these therapies.
Additionally, we think HIF-mediated immune evasion is a major reason that the new immuno-oncology drugs—even though they can have dramatic effects in select patients—are not successful in most patients. Re-establishing just one immune pathway, as is the case with immune checkpoint inhibitors, is likely not enough to prevent cancer cells from evading the immune system. However, if we inhibit HIF activity, we can affect the expression of multiple factors that promote immune evasion; we think this strategy might be useful in sensitizing patients to immune checkpoint inhibitors.
What are some strategies for targeting hypoxia and HIFs for cancer treatment?
Whereas most cancer therapies target dividing cancer cells, which are generally the more oxygenated cells within the tumors, there aren’t any approved therapies that target hypoxic cells. We have spent the past 15 or so years trying to identify ways in which the HIFs promote cancer progression and to develop HIF inhibitors that might be useful to treat patients.
Our research has focused on understanding the process that leads to metastasis after various therapies. We have identified a large set of hypoxia- and HIF-induced genes that promote metastasis and have broken down the metastatic process into discrete steps to identify target genes responsible for each of those steps.
Based on this work, we believe that strategies targeting the cancer stem cells are extremely important. When a tumor recurs after initially responding to treatment, this is likely because a population of cells with stem cell properties survived the initial therapy and then came back to form the recurrent disease. Of course, targeting HIF-induced immune evasion pathways is also going to be critical to prevent development of the lethal cancer phenotype.
What are the latest advances in targeting HIFs for cancer treatment?
There have been some encouraging clinical results with an inhibitor of HIF-2. The inhibitor, PT2385, binds directly to the HIF-2α subunit and prevents its dimerization with HIF-1β, which is a necessary step to activate expression of HIF-induced genes. This drug is currently being tested in patients as a treatment for kidney cancer, where HIF-2α plays a particularly important role. The phase I trial with this drug showed some promising results in patients with advanced kidney cancer.
It is quite amazing that what started out as very basic research on protein structure quickly led to the development of this drug that may be useful in treating cancer. For many years, the dogma in the pharmaceutical world seemed to be that the HIFs were not druggable targets because of their nuclear localization. These studies provide proof of principle that targeting the HIFs is a bona fide strategy for cancer therapy.