What is CRISPR-Cas9 and How is it Used in Cancer Research?
The 2020 Nobel Prize in Chemistry was awarded to Fellows of the AACR Academy
Emmanuelle Charpentier, PhD, and Jennifer A. Doudna, PhD, for their groundbreaking development of the CRISPR-Cas9 genome editing system.
This technology allows researchers to accurately delete, add, or modify specific regions of the genome and has proven to be valuable across various fields of research, including cancer research.
What is the CRISPR-Cas9 system?
CRISPR-Cas is a widespread, naturally occurring DNA-cutting system in bacteria and other microorganisms that was initially identified in the 1980s. More recently, in the early 2000s, it was discovered that the CRISPR-Cas system in these organisms serves as a defense mechanism against viruses. In bacteria, invading viral DNA is fragmented into short segments and incorporated into the host genome, organized in arrays known as “clustered regularly interspaced palindromic repeats,” or “CRISPR”. If the same virus infects the bacteria in the future, RNA molecules produced from the CRISPR array will guide a DNA-cleaving protein called Cas (which stands for “CRISPR-associated”) to the corresponding viral DNA. Here, Cas will function as a type of “genetic scissors,” cutting the viral DNA into many pieces, thereby leading to its destruction.
In a landmark paper published in Science in 2012, Doudna and Charpentier demonstrated that this naturally occurring process could be repurposed into a genome editing tool. Similar to the process in bacteria, CRISPR-Cas9-mediated genome editing relies on an RNA sequence—called a “guide RNA”—to direct the Cas9 protein to the correct DNA site. Once the Cas9 protein is guided to the correct genomic site, it will digest the DNA, thereby deleting the targeted gene.
In bacteria, the guide RNA forms naturally in response to viral infection, but in the case of genome editing, the guide RNA is carefully designed by the researcher to target a specific region of the genome. In some studies, researchers design their CRISPR-Cas9 assay to simply delete a gene, while in others, they insert a different gene in the place of the one that was deleted. Modifications that inactivate the DNA-cleaving activity of Cas9 are also sometimes used to enable the Cas9 protein to bind DNA, but not digest it. This binding can inactivate the target gene without deleting it.
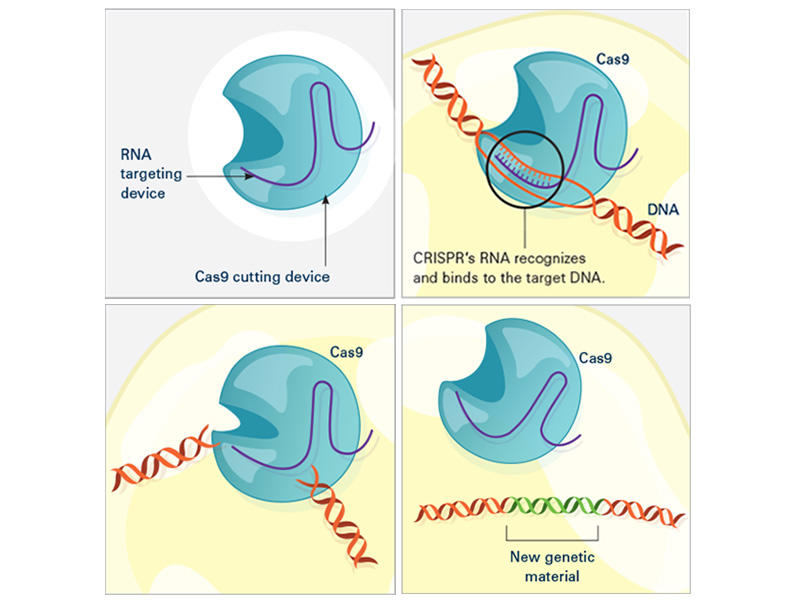
Compared to other genome editing tools, CRISPR-Cas9 is cheaper, faster, and more accurate. Unlike prior methods, such as zinc finger nucleases and TALENs, the CRISPR-Cas9 method requires only a short stretch of RNA to target a genomic site, making it easier to design and employ. Furthermore, the increased accuracy of CRISPR-Cas9 compared to these other methods reduces the likelihood of off-target effects, making it safer for potential clinical applications.
How is CRISPR-Cas9 used in cancer research?
Since its inception, the CRISPR-Cas9 technique of genome editing has been used in a myriad of basic cancer research studies. By deleting, inactivating, or otherwise modifying specific genes in cultured cells or in animal models, researchers have been able to study the role of these genes in cancer development and progression, providing insight into how to prevent or treat certain cancers.
In some cases, CRISPR-Cas9 has been used to study the role of a specific gene of interest, such as in a study published last month in the AACR journal Cancer Research. In this study, researchers used the technique to understand how inactivation of the BRG1 gene, which is commonly mutated in non-small cell lung cancer, contributes to cancer progression. They utilized CRISPR-Cas9 editing to delete BRG1 in lung cancer cell lines and found that BRG1-deficient cells had increased replication stress and were sensitive to pharmacologic inhibition of the ATR checkpoint protein, revealing a mechanism of cancer progression and a potential therapeutic opportunity for the treatment of lung cancer.
An additional application of CRISPR-Cas9 is in genetic screens, where hundreds of genes are deleted individually to identify key regulatory proteins, potential drug targets, and/or to test responses to experimental drugs in a relatively quick manner. This approach was exemplified by another study published last month in Cancer Research, which utilized a CRISPR-Cas9 genetic screen to identify potential therapeutic targets for lung cancer. By individually deleting over 500 different genes that interact with or modify chromatin, researchers determined that deletion of the histone chaperone nucleophosmin 1 significantly reduced tumor progression in vitro and in vivo, thus identifying a potential drug target.
Can CRISPR-Cas9 be utilized in cancer treatment?
In addition to its widespread use in basic cancer research, the CRISPR-Cas9 system is also being explored as a way to improve cancer treatment. In a keynote address delivered at the AACR Virtual Special Conference: Tumor Heterogeneity, immunologist, oncologist, and Fellow of the AACR Academy Carl June, MD, described the potential utility of CRISPR-Cas9 in adoptive cell therapy, a form of cancer therapy that uses a patient’s own immune cells to destroy cancer cells.
In adoptive cell therapy, T cells (a type of immune cell found in blood) are extracted from the patient, engineered to express a T-cell receptor that will recognize the patient’s cancer, multiplied, and reintroduced into the patient. Adoptive cell therapy has been successful for the treatment of many blood cancers in both adult and pediatric patients; however, several challenges remain to using this therapy widely, including cancer-mediated activation of immune checkpoints.
Immune checkpoints constitute a normal regulatory process that prevents overactivation of the immune response and subsequent damage to normal tissue. Immune checkpoints are activated by the binding of certain receptors (PD-1 or CTLA-4) on the surface of T cells to their ligands (PD-L1 or B7, respectively) on target cells.
Some cancer cells express high levels of the PD-L1 or B7 ligands even in the absence of immune activation, allowing cancer cells to activate immune checkpoints, turn off the T-cell response, and circumvent the immune system. This presents a problem for adoptive cell therapy: While the engineered T cells can recognize the patient’s cancer, they are inactivated before they can mount an antitumor immune response.
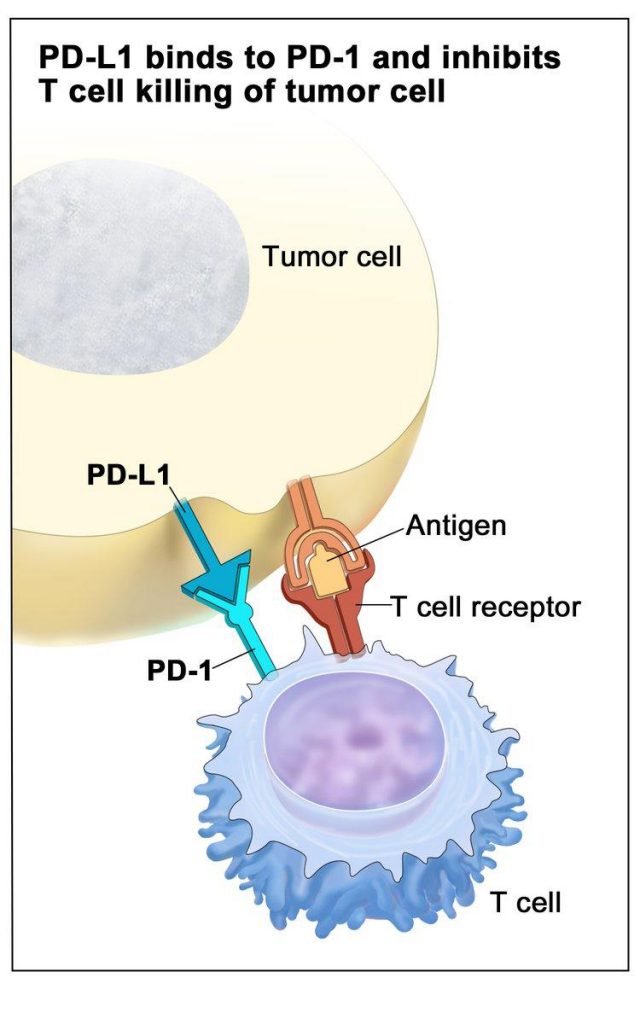
One approach to addressing this challenge is to utilize CRISPR-Cas9 to delete the gene expressing the PD-1 receptor in the patient’s T cells. Researchers hypothesize that the absence of the immune checkpoint would allow the T cells to remain active. Various studies have demonstrated that this strategy improves responses in preclinical models, but the feasibility of this approach in patients remains unclear.
In his presentation, June discussed results from the first-in-human trial of CRISPR-Cas9-modified T-cell therapy, which were published earlier this year. In this study, June and colleagues used CRISPR-Cas9 to delete from T cells the gene expressing PD-1, as well as genes expressing endogenous T-cell receptors to prevent competition with the engineered T-cell receptor. The CRISPR-Cas9-modified T cells were administered to three patients with advanced cancer, including two patients with refractory advanced melanoma and one patient with refractory metastatic sarcoma. Stable levels of the T cells were detected in the blood of all three patients several months after infusion.
None of the patients experienced any serious adverse effects from the treatment, and there was no evidence of autoimmunity or cytokine release syndrome, a potentially lethal side effect that can be associated with adoptive cell therapy. The engineered cells were able to recognize and destroy cancer cells from all three patients, and the treatment led to stable disease in two of the patients. The third patient had a mixed response, which included a 50 percent reduction of one of their tumors but progression of other tumors.
Gene expression analysis of the infused cells in one patient showed that the engineered T cells had increased expression of genes associated with T-cell memory, but not T-cell exhaustion, suggesting that deletion of PD-1 may have prevented the activation of immune checkpoints.
June concluded that editing of T cells using CRISPR-Cas9 technology was safe and feasible for cancer treatment. Further research will be required to determine the potential of this approach to prevent immune checkpoint activation and improve clinical responses.
By discovering a primitive bacterial defense system and transforming it into a powerful genome editing tool, scientists have greatly expanded the possibilities of research in diverse realms of science and medicine. As additional applications of CRISPR-Cas9 are conceived, studied, and applied, the impact of this technology is sure to grow.
Hi. Nice Article. Keep sharing more information related to CRISPR Technology !!