Annual Meeting 2022: How do Tumors Evolve to Resist Therapy?
The story is familiar: A patient is diagnosed with cancer, they begin treatment, and, after a few months, they receive word that their tumor is shrinking. Thrilled, they continue their treatment with a renewed sense of hope, until another scan reveals that the tumor has stopped shrinking. In fact, it is growing again.
Cancer has an unsettling ability to respond to external pressures, including treatment. Often, cancer cells have mutations that keep them dividing even when the DNA hasn’t been properly checked for damage or replication mistakes. This “error-prone” process of cell division makes it easier for cells to acquire new mutations, including those that may improve tumor growth or render therapy ineffective. These cells have a survival advantage in the tumor environment, which helps the cancer evolve.
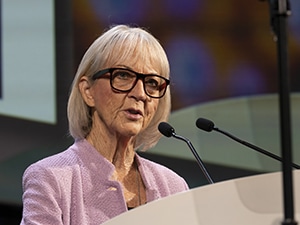
“Nothing in cancer makes sense except in the light of evolution,” said Anna D. Barker, PhD, FAACR, Chief Strategy Officer at the Ellison Institute for Transformative Medicine at the University of Southern California, paraphrasing Theodosius Dobzhansky, a pioneer in the field of evolutionary biology.
Barker chaired the plenary session titled Tumor Evolution and Therapeutic Resistance at the AACR Annual Meeting 2022, held in New Orleans April 8-13. This was the first Annual Meeting plenary session held on this topic and was developed by the newly formed AACR Cancer Evolution Working Group, of which Barker is co-chair elect.
In her opening remarks for the session, Barker encouraged the audience to think critically about the ways cancer evolution could inform all stages of research from the bench to the bedside. “I believe that cancer evolution represents one of the most critical areas of cancer research that can truly transform patient outcomes,” she said.
Mechanisms Driving Tumor Evolution
Normal cells possess many checks and balances that prevent them from growing out of control. To evade these preventative mechanisms, a cell must acquire multiple mutations, which it then passes onto its progeny to form a tumor. If any cells in the developing tumor acquire additional mutations, the tumor becomes a mixture of different subclonal populations.
Though many researchers have investigated how the diversity of those populations shapes tumor progression, information about how a cancerous clone develops from normal cells is limited.
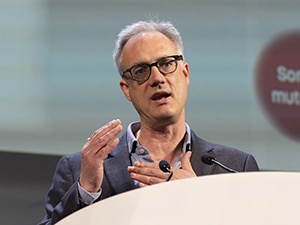
“If we really want to understand what goes on in the early stages of cancer evolution, we need to be looking at normal tissues,” said Peter Campbell, MBCHB, PhD, FAACR, head of Cancer, Aging and Somatic Mutation at the Wellcome Sanger Institute in the United Kingdom. Campbell studies clonal mutations in the normal breast tissue of individuals with and without breast cancer, in order to understand how mutations accumulate over the course of an individual’s life.
By sequencing DNA from dozens of microscopic samples isolated from the breast tissue of a single individual, Campbell and colleagues can use the abundance and relative location of mutations to construct a phylogenetic tree that describes how the mutations are related. The researchers can even pinpoint periods of prolific mutation and trace them back to significant events in breast development—such as puberty, pregnancy, or menopause.
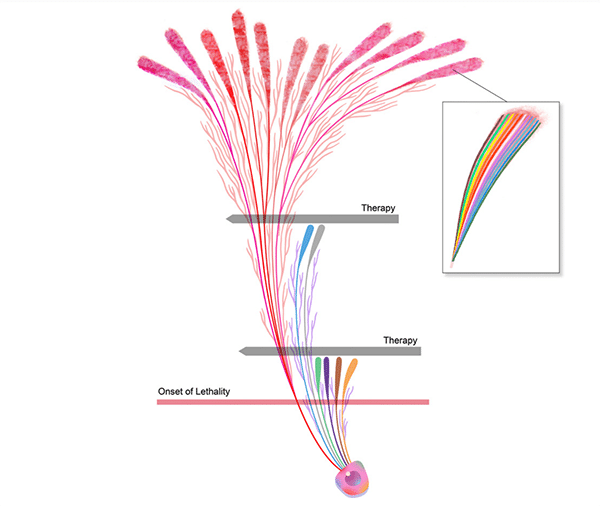
Understanding how and when driver mutations accumulate can help researchers better decipher the processes leading to cancer and whether those processes differ in other tissue types. In breast cancer, for example, mutations that occur after cells become malignant are driven by different mechanisms than mutations that occur in normal breast tissue. “The mutation burden is always orders of magnitude higher in the cancer than in the matched normal tissue,” Campbell explained. “That increase in mutational burden is caused by cancer-specific mutational processes, which isn’t what we see in all tissues.”
Decoding the process of cancer evolution in blood cells requires a different approach due to the inherent differences between blood and solid tissue renewal. New blood cells are produced by hematopoietic stem cells which maintain the unique ability to produce several blood cell lineages. When differentiation of daughter cells through these lineages is prevented, or when there is a shift in the proportion of differentiated cells being made, cancer can occur.
“The goal is to understand what regulatory processes in stem and progenitor cells are hijacked in malignancy and contribute to increased severity and evolution of the disease,” said Emmanuelle Passegué, PhD, director of the Columbia Stem Cell Initiative at Columbia University Irving Medical Center. The ultimate objective is to target the deregulated processes, she noted.
Passegué described one such process, a program of “emergency myelopoiesis,” or a shift toward the production of more myeloid cells, a type of immune cell. While this process is typically activated to boost myeloid cell numbers in response to infection or other stresses, it can be co-opted to fuel cancer development.
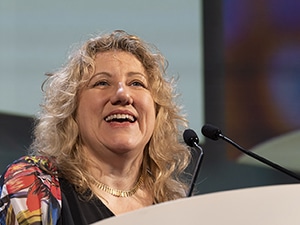
Emergency myelopoiesis can be activated by factors such as inflammation, stress, and signaling from certain cytokines. If these processes go awry, a resulting increase in myeloid cells could drive precancerous changes such as myeloproliferative neoplasms and myelodysplastic syndrome, or eventually acute myeloid leukemia. Aging, Passegué noted, can drive inflammation and damage of the bone marrow, increasing the risk of such changes.
Passegué and colleagues are working on ways to dampen emergency myelopoiesis and rebalance the differentiation cascade. They have found that, in preleukemia, myeloid progenitors called MPP3 cells secrete cytokines that stimulate themselves to continue driving myeloid cell production. In preleukemic mice, genetic knockout of the secreted factor Gal3 prevents emergency myelopoiesis and delays the onset of leukemia.
Strategies for Studying Tumor Evolution
Tracing the evolutionary lineage of cells from human samples is a relatively new feat enabled by recent technological advances, such as the ability to profile DNA, RNA, and epigenetic marks in individual cells rather than a bulk population.
Nicholas Navin, PhD, a professor of genetics and bioinformatics at The University of Texas MD Anderson Cancer Center, explained how single-cell technologies are being used to study cancer evolution. He and his team have developed computational methods that use single-cell DNA sequencing to characterize gains and losses in copies of a DNA sequence and to map the evolutionary history of different subclones.
“Single-cell DNA sequencing technologies can be used to understand the clonal composition of a tumor,” said Navin. “You can use this data to understand the order of mutations that occur throughout tumor growth and how populations expand in the context of different time point samples, such as primary and metastatic samples or pretreatment and posttreatment samples.”
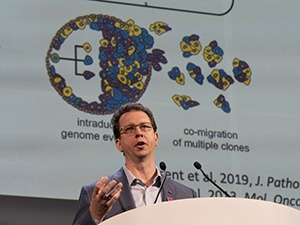
Navin and his team used these technologies to investigate the mechanisms by which DCIS, a noninvasive form of breast cancer, progresses to invasive disease. After developing a method to perform single-cell sequencing on archived tissue from a biobank, the researchers analyzed samples from 10 DCIS patients who experienced tumor progression. Although the invasive tumors had significantly more subclones than the DCIS lesions, in eight of the 10 patients, a single DCIS subclone kickstarted the invasive tumor.
Navin is also working to use these methods to understand the cell types, cell states, and evolutionary lineages of normal breast tissue, as part of the Human Breast Cell Atlas initiative. He and his colleagues are especially interested in characterizing cells in the breast microenvironment, such as immune cells, fibroblasts, and blood vessels.
“We think this will serve as a Human Genome Project-like reference for studying DCIS, breast cancer, and other breast diseases,” Navin said.
Defining different subclones within a tumor can provide important information about tumor metastasis as well, according to Charles Swanton, FRCP, PhD, FAACR, a senior group leader at the Francis Crick Institute in the U.K. and co-chair of the Cancer Evolution Working Group. He and his colleagues are studying tumor evolution from primary lung cancer to metastasis in a cohort of 421 patients from the TRACERx clinical trial.
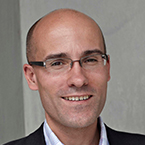
“We wanted to establish the origins of the lethal metastatic subclone or subclones and help distinguish metastatic-competent from incompetent subclones in the same tumor,” Swanton said.
The cohort contains over 1,600 samples taken from different regions of the primary tumors, allowing the researchers to create a spatial map of the subclones found in each tumor. They found that some tumors have a dominant subclone and others contain a fairly even mixture of multiple subclones. Dominant subclones, they found, were associated with a significantly shorter disease-free survival.
Of the 421 patients, 129 experienced a metastatic recurrence, resulting in 227 samples from different metastatic regions. When Swanton and colleagues sequenced the metastases, they found that if the matched primary tumor contained a dominant subclone, it was usually the clone that seeded the metastasis.
Swanton and colleagues sought to determine defining characteristics of these metastatic subclones, such as when they developed during the course of tumor evolution. The researchers found that around 65 percent of the time, the metastatic subclone evolved later in tumor development, after the tumor had 100,000 cells or measured 1 mm in diameter.
They also wanted to evaluate whether the metastatic subclone could be identified in circulating tumor DNA (ctDNA) prior to metastasis. In the majority of cases, the subclone that went on to seed a metastasis was present in a significantly higher proportion of ctDNA than other subclones.
Nevertheless, Swanton also found that some metastatic lung cancers resulted from multiple subclones. Patients with polyclonal metastases were more likely to experience metastasis outside the chest cavity and, on average, had a poorer prognosis.
Biomarkers and Intervention Strategies
While it is important to understand the underpinnings of tumor evolution, it is equally important to translate this knowledge into clinical interventions. Christine Iacobuzio-Donahue, MD, PhD, director of the Center for Pancreatic Cancer Research at Memorial Sloan Kettering Cancer Center, is working to develop ways to battle tumor evolution in pancreatic cancer before it can metastasize.
Although clinical trials are often geared toward treating patients with metastatic disease, around 30 to 35 percent of pancreatic cancers are diagnosed at stage 3, Iacobuzio-Donahue explained. At this point, the tumor cannot be safely removed with surgery, but it has not yet spread to other sites.
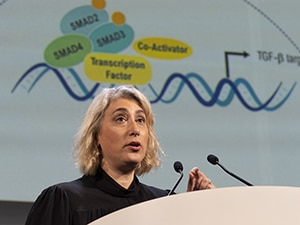
“This patient population represents a tremendous opportunity to really move the needle on the survival of this disease,” Iacobuzio-Donahue said, adding that around 22,000 patients in the U.S. will be diagnosed with stage 3 pancreatic cancer this year. “We’re talking significant numbers of patients to make a difference for.”
The challenge for this patient population, she explained, is to predict which patients will respond to chemoradiation therapy and which patients will experience progression. In the search for potential biomarkers, Iacobuzio-Donahue and colleagues identified inactivation of the protein SMAD4, a member of the TGFβ signaling pathway, as a strong predictor of progression.
Curious about the mechanism of SMAD4 inactivation, the researchers performed whole-exome sequencing on multiple regions of primary tumors and metastases from 35 patients. They found that inactivating mutations in SMAD4 were often subclonal, developing around the time of invasion. SMAD4 also wasn’t the only TGFβ gene mutated in this population; the study revealed a large subset of patients with mutations in the TGFβ receptor TGFBR2.
This suggested a strong selection pressure driving survival and metastasis of cells with TGFβ pathway mutations. “When you see a really dramatic change, look to the microenvironment for clues about why that change is selected for,” Iacobuzio-Donahue said, quoting a friend who studies species evolution.
Iacobuzio-Donahue and colleagues examined the tumor microenvironment and found that stroma with robust TGFβ secretion correlated with decreased tumor growth and metastasis. This supported the idea that sustained TGFβ signaling between the stroma and the tumor is important to prevent metastatic spread.
Although Iacobuzio-Donahue admits that developing clinical strategies to prevent or reverse loss of TGFβ will take time, these data present several avenues for further research toward that goal. “We are continuously trying to stay focused on the evolutionary features that are clinically relevant and that we can potentially act on,” she said.